Shock Tuning Payoffs
Autocross to Win has been out for a few years now, and it generates a slow but steady stream of e-mailed questions.
The most popular question is "Will you build me a set of shocks?" (No. I have neither the time nor the equipment to do so)
The second most popular question is something along the lines of "If I do all this stuff, how much faster will the car be?"
I quite honestly cannot answer that question, (there's too many known unknowns). So my usual response is "I can't answer that question". And that response is not very popular. It sounds like a dodge or an evasion.
That people think I'm evading the question is very frustrating - because one of the key themes of A2W is that without doing the measuring and modelling of your specific car nobody is capable of predicting its performance, so how should I be able to do it? A reader disappointed with the "I don't know" answer clearly hasn't learned the central lesson, and that in turn means that I have failed somewhere as an author and teacher.
But also vexing is that there are plenty of counterexamples of people who have not taken the analytical approach to their car setups who are, notwithstanding, very successful in competition. These are drivers who are unquestionably, by virtue of not optimising their tuning through the analytical approach, leaving some degree of theoretical performance "on the table". Given the close nature of competition, how can they be having the success that they are with all of this latent performance left untapped? How much of a difference is there really between a car that is fast because of its setup, and one that is fast in spite ofits setup?
I have thought long and hard about how to predict the degree of payoff associated with suspension and shock tuning, and I think I have finally figured out a way to conceptualize what is going on:
To start, let's make the following assumptions:
- You have the load sensitivity curves for your tires (how grip changes with normal load);
- The traction circles for all four tires are the same, and they are all round (not oval as is usually the case);
- All tires are always at optimum temperature, pressure, and camber angle;
- The car is set up with a static weight distribution that is 50:50 front to back, and 50:50 side to side (that is, all the weights on all four tires are the same with the car sitting still);
- The car is sprung as softly as possible while still keeping control of dynamic camber, roll centre location/propagation, and the like;
- The location of the CG, the track and wheelbase, and both front and rear roll centre locations are all fixed (this isn't too far from the truth for any production-based car);
- We have a way of directly measuring the grip at the contact patch of each tire; and
- We have access to RoboDriver™; a driver who can drive the car flawlessly with no human error. (Mark D. makes an acceptable substitute).
None of these assumptions make any material difference to the example; this is just a way of clamping some of the variables at first.
OK, so with these assumptions in place, take the weight of the car, divide it by four, and use that value as the lookup on the tire load sensitivity chart. Take the resultant grip value, multiply that by four, and what you have is the maximum level of grip that the car can ever have (barring aerodynamic effects). That's it. Four equally loaded tires in their respective "happy place" with regard to camber angle, temperature, pressure etc produce the maximum level of grip that the car can ever produce. Nothing (again, aside from aero downforce) can increase this value.
Now let's take that car and RoboDriver™ out to a test course, consisting of a single 180° turn laid out on perfectly smooth and flat high-grip concrete. The car will start from a standing start, negotiate the turn, and come to a complete stop right at the finish line. RoboDriver™, being RoboDriver™, can do this flawlessly and the line he chooses will be optimum.
If you graph the instant grip value as it changes with time (the grip measurements at all four contact patches added together) as the car makes its run, you will see that grip changes as the car accelerates away from the start, negotiates the turn, and comes to a stop - and that the instant grip level is always less than the maximum value. This is due to the inescapable fact of weight transfer. Because of tire load sensitivity, any weight transfer away from four equally loaded tires reduces grip, so with a car that starts out equally loaded at rest produces maximum grip at rest, and will only lose grip as weight moves around in response to driver inputs.
You should also see that the instant grip curve with respect to time is fairly smooth. It moves around, but there are no hops or dips in the curve that indicate sudden changes in grip level.
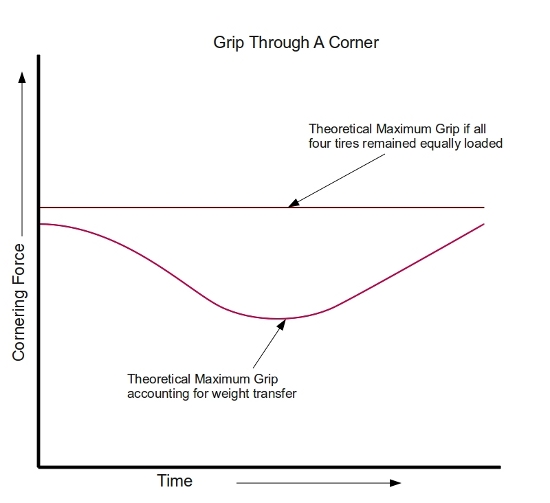
This curve represents the maximum level of grip possible through this turn, which means it also represents the quickest path through this turn.
Now we take a "real" race car, and throw away all the assumptions save the 50:50 left/right, front/rear static weight distribution assumption. Put RoboDriver™ in the car, and repeat the test. Any deviation of the grip curve away from the ideal curve represents lost time.
These deviations will take one of two forms:
- An "offset" below the optimum grip curve, but in parallel to it; or
- "Dips", where there is a large change in a short period of time.
An "offset" is an incremental difference. For example, the car might be slightly oversprung compared to what is needed to keep dynamic camber etc. under control. If that is the case, than the contribution to weight transfer from the springs will be slightly higher than optimum, and grip will be correspondingly lower in all cases where lateral weight transfer is happening. Slightly oversprung will produce a slight change; heavily oversprung will be a large change.
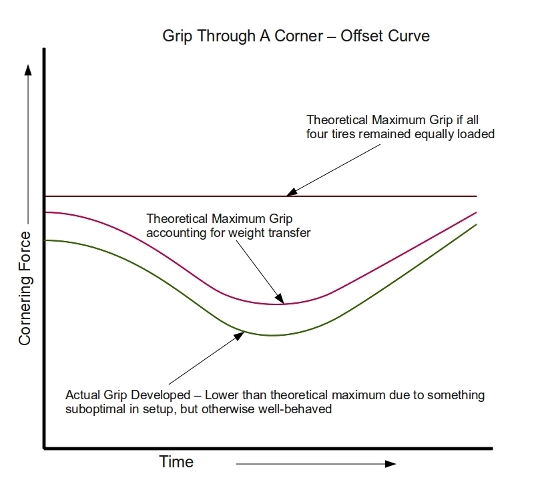
Assuming that the setup is reasonable though, the deviation from optimum is probably not all that much. Little increments can add up to big ones, but it turns out that it can be very hard to really screw up a car so that grip is way down all the way through the curve. There are unquestionably gains to be made here, but they are, for the most part, incremental.
"Dips", on the other hand, are bad news.
A "dip" represents a sudden loss of grip - and in this example, we are looking at the sum of the grip from all four tires. In reality, a "dip" probably comes from a loss of grip on the most highly loaded tire on the car, or the second most highly loaded - and that means a change in attitude, not just a general slowing down.
For example, if the outside front tire suddenly loses grip, the nose will step out. If the outside rear loses grip, the rear will try and come around. If both lose grip, the car will bound sideways, and if any one tire suddenly loses grip while braking, the car can quickly start locking wheels and sliding.
Any "dip" in the grip curve represents a condition where the car will suddenly do something untoward, and the driver will have to make a control input to get the car back on line. That, in of itself, loses some time, and depending on the driver and the line, it may not be recoverable. That "dip" might represent a spin, or a cone being struck, or a compromise of the line into the follow-on corner. It means an immediate loss of time as the "dip" is recovered, and it may mean a further loss in time as the consequences of the recovery are carried forward.
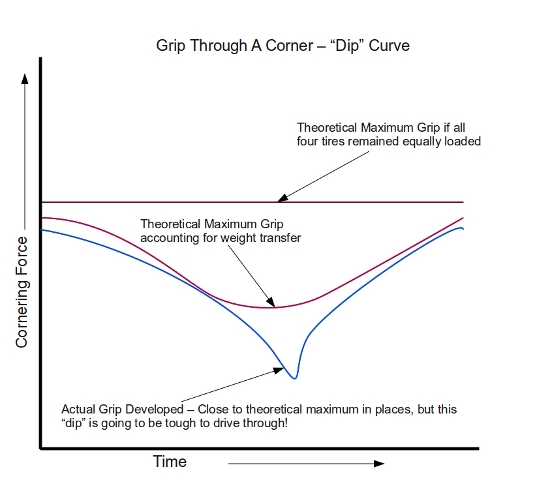
An example is required:
The competition surface at Grissom AFB in Peru, Indiana, is a giant concrete B-52 staging pad. Because the pad is so large, it tends to collect a good deal of water when it rains. Because concrete does not absorb water very well, the sub-pads are angled towards each other to encourage the water to run off the face of the sub-pads into shallow "ditches" formed at the intersection of two sub-pads, and these "ditches" are further angled to direct water towards the storm drains.
Driving over these "ditches" in a B-52 moving at 10 km/h is barely noticeable. Crossing one at 100 km/h in a race car will get your attention. Having one of these bisect a corner really plays havoc with one's day.
I was at a Peru race where there was a shallow 60° corner joining two fast sections, and this corner had one of these "ditches" crossing it right after the corner entry. The corner itself was gentle enough that most cars were capable of taking it flat out, and because this corner connected two fast sections, there was a LOT of time to be had in making that corner flat out. Anything slower than flat out meant carrying less speed into the follow-on fast section, so screwing up that corner cost you twice - once in the corner itself, and again in the follow-on section.
Watching cars go through this corner was very entertaining - and very instructive - because most cars that day had a "dip" in their grip curve where that bump intersected that curve. Cars were bounding around, stepping out, and generally coming unglued as they encountered the bump.
The instructive part was watching second and third runs as drivers attempted to deal with the bump:
- Some just slowed the corner down to minimize the impact of the grip "dip" - these drivers gave up time both in the corner and the follow-up section, and were slow.
- Some gambled on their ability to capture the car after it got upset, keeping the same line that they would if there had been no "dip" in their grip curve. These gambles rarely paid off, as the follow-on manoeuvre needed to save the car usually exceeded the grip available at that point in the curve. These guys typically spun or hit cones, but if they got lucky and made it, that would be a fast run. Watching the shenanigans was entertaining, but this was a low percentage option. Most of these guys wound up slow.
- But a few drivers had taken note of what the car was doing when it encountered the bump, and changed their line on entry so that the car's reaction to the bump put them on the ideal exit line. These drivers gave up some time in the corner because the "dip" in the grip curve forced them off the ideal line. But by using the car's reaction to the bump as part of the cornering strategy, they minimized the amount of time lost and accordingly optimised the follow on section as best they could. These guys were fast, and they were consistently fast.
Of the three approaches, option number three was the way to do it.
My approach was entirely different.
My car, at that time, had been through several iterations of valving based on suspension speed histogram analysis and measuring and modelling the suspension. While not entirely perfect, it was very, very good.
It did not have a "dip" in the grip curve at that bump. The suspension, specifically the shock valving, was capable of handling that bump with no appreciable loss of lateral grip.
So I blasted through that turn, on the ideal line and under power, and put about a second and half on my competition.
In retrospect, when you go back and examine my results, you can see that (once I adopted the shock tuning strategy used in A2W), the bumpier the course was, the faster I was relative to my competition. Billiard-smooth tracks like Toldeo didn't put cars into conditions where their grip curves had "dips" in them, so the drivers had no need to either slow down or work miracles to accommodate those "dips". To some extent, there is also a correlation with certain course layouts, although that is much harder to capture in the data (where "bumpiness" is easily captured through suspension position sensors).
So imagine a course that is infinitely long. It consists of one of every kind of corner on every kind of surface with every kind of bump in it. Imagine the grip curve that results from RoboDriver™ driving your car through this course. Any corner where there is a "dip" in the grip curve represents a condition that will potentially punish your time. You may, during your career, encounter this condition once, or many times - or never. Depending on what kind of driver you are, you may be able to work around the "dip" in the grip curve - or not.
But suspension tuning optimization as detailed here eliminates (or at least greatly reduces the frequency of) these "dips" - meaning that the car is overall easier to drive, and thus faster on average, even if the maximum grip level is never increased.
You also should see some incremental improvements to overall grip levels, and those increases to maximum grip will help too. But by far the biggest improvement is the elimination of bad habits in the car.
Another observation:
Of those drivers who are capable of incorporating their cars' bad habits into their runs and thus minimizing (but not eliminating) the consequences of those habits - I'm convinced that most of them are not consciously aware of what they are doing. For them, it is "natural" that cars should do the things that they do, and the idea that there is even a problem to tune away seems to be utterly foreign to them. Many is the time that I have driven someone else's car that has a huge and (to me) glaring bad habit, and when I pointed it out to the driver, they were astounded that I had that opinion. I have said it before, and I'll say it again - really good race car drivers adapt very, very quickly to even the craziest setups and "wonky" can very quickly become "normal". The very best can make use of a car's bad habits and will minimize their effects - and will do so without even thinking about it.
Paradoxically, this can make discussing car setup with the truly talented somewhat counterproductive.
Following an analytical approach to suspension tuning will eventually provide the performance margin required to beat these guys, because the elimination of "dips" in the grip curve plus the incremental improvements will eventually carry the day, but there is still a requirement to drive well. A poor car driven superbly will almost always beat a superior car driven poorly.
Happily, if you are doing it right, tuning the suspension makes the car easier to drive well. It is much less about raising the absolute limits of the car, and more about bringing the car into the talent range of the driver.
The other aspect of this is that if one of these real talents ever decides to do the work and properly prep their car... they will be VERY fast. But human nature is on the side of those with less driving talent but a stronger work ethic. If a talented guy is winning now, what's the motivation to do the prep work?
There is one other aspect to making the car easier to drive - it makes it easier to learn how to drive, which means that someone whose talent start state is lower can develop their skill to a higher level. Talent can be trained, but the surest way to bring learning to a screeching halt is to have the car be overly punishing to mistakes. A more forgiving car is conducive to learning, and learning is conducive to speed.
All of this means that it is very difficult to predict an absolute value for performance improvement from a program of analytical suspension tuning. It's not like power and drag racing, where one can predict (with very high accuracy) how much faster a given power increase will make the car. It is, however, clear to me that there are revolutionary gains waiting to be had by eliminating "dips" in the grip curve, incremental gains to be made in raising the grip curve to be closer to optimum, and incremental to revolutionary gains to be made in helping the driver learn his trade. All of which make the time and money invested highly worthwhile.
How about the Street?
Another common question is "How much does all this matter for a street car?" (usually asked in the context of somebody who has just dropped a couple of grand on a set of Teins or some similar off-brand T00ner shock).
The paradox of a street car is that its operating environment is such that you are only visiting the portion of the performance envelope where shocks truly matter only irregularly, if ever. This makes a street car more than an little insensitive to suspension changes, particularly shock valving changes.
This is true even of the car as it rolls off the dealer lot. Consider how much the natural frequency of the suspension changes between a little guy driving with a nearly empty tank, and a big guy and three big passengers plus a full tank of gas and all their luggage - easily a 1000 lb swing in sprung mass with no physical changes to the car. Sports cars are a little more resistant to this by virtue of having less seats and smaller luggage spaces, but nevertheless, a street car has a much less controlled setup environment and very rarely is ever operating on the limit of grip.
The "paradox" portion of this is that the insensitivity of the car to suspension setup means that shit can perform (within certain limits) every bit as well as super high end stuff. If your Corvette ever only travels to and from your house, your office, and the golf course, it can be very difficult to tell the difference between a Monroe Sensi-Trac and a triple-adjustable Penske.
I'm convinced that this insensitivity is THE reason why so many of the Japanese shock manufacturers (and the Chinese clones of same) are valved so strangely. You can't "feel" a properly-valved shock, but if you've just bought some aftermarket performance shocks, you expect to feel some sort of change. It takes a very large change in shock force to really be detectable by an average driver so if you want the customer to feel like his money bought something, you have to valve the shocks with some pretty outrageous forces.
One of my real eye-openers was my personal daily driver; a 1993 Dodge Stealth. This car comes equipped with a computer-controlled "active" suspension. Each shock has a small electric motor attached to a rebound bleed adjuster, and the motor has three positions - hard, medium, and soft. A computer is attached to each motor, and a couple of sensors (accelerometers, steering angle sensor, etc) feed the computer with inputs. Under the right circumstances, the computer will adjust the rebound force on the shocks harder (the default position is full soft) in order to prop up that end of the car. In effect, it uses the Stock class strategy of using excessive shock rebound force as a "fake spring", but on demand rather than all the time. In some ways, it is almost laughably crude, but it is also completely effective. It's a very clever hack.
But it also has a user control - a "Sport" switch. In "Sport" mode, the computer just sets all four shocks to full rebound and leaves them there. And "full rebound" is actually pretty stiff.
What makes this an eye-opener is that by toggling the "Sport" switch one can change the rebound forces on the car by an enormous amount and compare how the car changes immediately. You don't have to stop the car, twiddle an adjuster, and get going again; you can experience it in real time. And this isn't a tiny tweak, the force difference between "Tour" and "Sport" is very large.
Setting the car into "Sport" mode immediately changes the ride quality. The car goes from ever-so-slightly floaty to borderline jiggly. "Sport" mode is actually slightly uncomfortable. But - and this is important - I find that I adapt to it very quickly and "Sport" starts to feel "Normal" within a few seconds.
If you can find a similar car, it can be very instructive to feel this for yourself.
There are a couple of key lessons here:
- The first is that a street car will be very tolerant of wide swings in setup. Bad behaviour will only manifest itself during normal use with very, very extreme setups. The "so what" here is that if your street car feels extreme during normal use, something is probably very, very wrong.
- The second is that you are only going to experience the true benefits (or punishments) from your setup at the limit of grip. A race car should be operating here exclusively. A street car should never be operating here except the split-second of an emergency avoidance maneuver. This means it is going to be tough to test.
- The third is that long-term reliability is going to be more important than ultimate performance. You aren't going to have hot tires on a perfect road surface and perfect conditions; you are going to have cold tires on a wet and sandy road with broken pavement when three moose jump out in front of you and you have to slalom them (true story!). Chasing the performance difference between a Koni Yellow and a perfectly tuned Penske isn't not just cost ineffective, it's not even possible.
- And finally, you adapt to new setups so quickly that you cannot count on your butt dyno to help you tune - it has to be done by the numbers.
OK then, so what to do with a street car?
- Put the car on the scales with just you and a nearly empty gas tank.
- Fill the tank, add your typical luggage load, and weigh the car again.
- Add a typical passenger, and weigh the car again.
- Fill the car with the largest load you think you'd ever reasonably carry, and weigh the car a final time.
- Run these numbers through the Dynamics Calculator. You now have a range of natural frequencies - and damping forces - that represents the operating environment of your car.
- Pick springs and damping forces that put the natural frequency of the car in its nearly empty state at 1.8 Hz to maybe 2.0 Hz MAXIUMUM. If you have to err, err on the soft side.
- Buy a set of Koni Yellows, Bilstein HD, or a similar good name-brand shock. Dyno them. If the shock forces fall somewhere in your operating range - good enough. If they don't then revalve with a view to 65% critical at the full empty state. But don't get wrapped around the axle here. Get it in the range, and you're done.