
Tech - The Case for a Single Chainring
One of the major technical innovations of 2010 was the introduction (first by SRAM with XX, then by Shinamo with Dyna-Sys) of the 10 speed rear cassette. With ten cogs on the rear, it becomes possible to have a very wide gear range with just a single shifter. This offers the potential to drop some weight off the bike (by eliminating the front derailleur, its shifter and associated cables, and the superfluous chainrings) as well as eliminate a potential source of failure (as front shifts are much more prone to dropped chains than rear shifts).
The cost associated with those benefits (less weight, reduced complexity) is a narrower range of gearing compared with setups that use multiple front chainrings. The question then becomes "how narrow is too narrow?" when applied to gear ranges.
Before we can perform this analysis though, we have to understand gearing and power.
The amount of force that a rider can apply to the crank spindle is called torque. Torque is a rotary force, and is calculated by multiplying the vertical force applied to the pedals by the length of the lever arm on the crank. All engines that generate a rotary force generate a torque; the higher the torque value, the stronger the twist. If you've ever used a torque wrench to measure how tightly a bolt has been twisted, you understand torque.
In the human case, because we intuitively have a feel for "how hard we are pushing on the pedals", one can conceptionally equate "torque" to "pedal force" and have the right idea, even though (strictly speaking) they aren't exactly the same thing.
Power is a measure of of energy; basically, the ability to produce torque over time. Because we spin the cranks by pedalling, it is convenient to think of time as being measured in crank revolutions per minute (RPM).
If you are capable of applying 1000 lbs to the pedals at 1 RPM, you are delivering a power of 1000lbs/min. Double the speed you spin the cranks to 2 RPM, and now you are producing power at 2000lbs/min and you have doubled your power output.
(Physics geeks - yes "lbs/min" isn't the proper unit for power; strictly speaking, it should be "foot-lbs/sec" and a constant to convert to horsepower (or do it in metric and get watts). But the concept as explained is correct and I don't want to get bogged down on the units)
Where power gets interesting is the fact that low torque impulses, done more often, can often produce more power than high torque impulses done less often. For example, if you can generate 100 lbs of force on the pedals, but do it at 60 RPM, then you are producing 60 impulses of 100 lbs per min, or 6000lbs/min - six times the power of someone producing 1000lbs of force at 1 RPM!
The other interesting aspect of power is that it is power that accelerates a vehicle. The raw torque value is only important when compared to the force required to overcome the various inertial forces and drag forces trying to prevent acceleration. So long as the torque is high enough to overcome these forces, more power means higher acceleration.
In other words, if we are trying to cover ground as quickly as possible, power is our friend.
Unfortunately, the power produced by any given engine is rarely a constant value. Most engines develop different torque values at different engine RPM values, and because power is just torque x RPM, this means we will get different power values at different RPM values too.
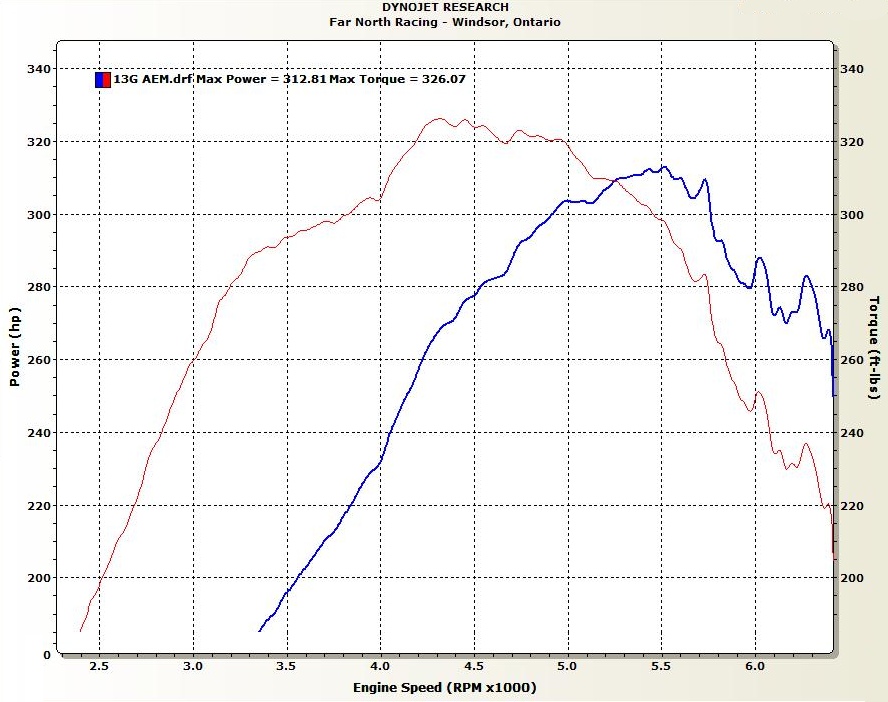
This is a dyno graph from my Dodge Stealth, showing torque and power. As you can see, the torque values change with changing engine RPM (the red curve) and so the power values change with changing engine RPM as well (the blue curve). In order to maximize acceleration, we want to keep the engine operating at the power peak for as much as possible.
Unfortunately, the wheels are connected to the engine, and accelerating means speeding the wheels up, which in turn means the engine cannot run at a constant speed. We therefore need a mechanism to change the ratio between the engine speed and the wheel speed.
If we could vary that ratio infinitely, then we could run the engine at its peak power RPM and just vary the ratio. Such a device exists; it is called a Continuously Variable Transmission (or CVT) and some cars (and most snowmobiles) have them. But most cars (including my Stealth) have a transmission with a fixed number of gear ratios in it, usually 5 or 6.
Given that our number of ratios is fixed, that implies we are going to have to run the engine in a range of speeds. It will start at a certain RPM, speed up to a second RPM, then, when the ratio is shifted to the next higher gear, the engine RPM will fall back to a lower value (based on the wheel speed at the shift and the value of the new gear ratio) and repeat the process with the next higher gear.
To maximize acceleration, we want to maximize the area under the power curve between each shift. This normally means shifting at some RPM point higher than the peak power RPM, so that the post-shift RPM and the shift RPM straddle the power peak RPM. Race cars have interchangeable gears inside the transmission so the engineer can select ratios to ensure this happens; production cars with fixed ratios require the driver to memorize shift points per gear in order to ensure the shift straddles the power peak.
The exact same process applies to a bicycle, except now the engine is the rider, the engine RPM value is the cadence value, and the transmission is the combination of the front and rear derailleurs, cogs, and chainrings. Just like the car, in order to maximize acceleration, we need to match the gearing of the "transmission" to the power curve of the "engine".
So what does a human power curve look like?
Well, we know that maximum torque happens with the bike stationary. The rider can push his entire weight and use the leverage from the handlebars to generate maximum force - think a BMX or 4-cross rider in the start gate.
We also know that there is a maximum cadence where the pedals are spinning so fast that it is all the rider can do to just keep the pedals spinning - think trying to spin the pedals on a fast downhill. At that cadence (or any faster) the rider can generate no torque at all.
For the points in-between, I don't have access to a power meter to see what the curve looks like. According to Bicycling Science, 3rd Edition (page 314) the torque values for the intermediate points lie along a straight line between the maximum and minimum points. It is not at all clear to me why this should be so, and I have to do some further reading to see if I can get an understanding at a biological level as to how this works... but for the time being, I'll go with it.
As a mathematical consequence of the relationship between torque and power, a straight, downward-sloping torque curve produces a parabola-shaped power curve.
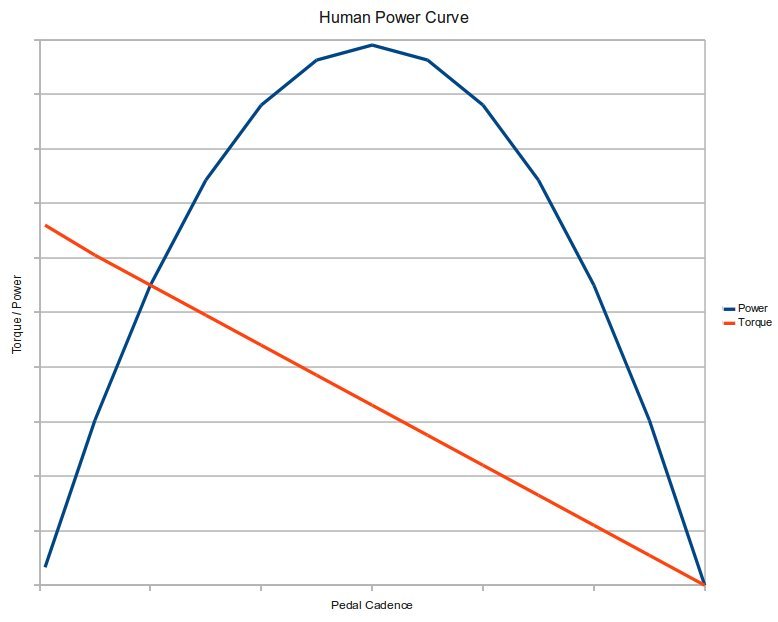
Which implies that humans make maximum power over a very narrow cadence range.
Interestingly, (and unlike cars) humans fatigue. The effect of fatigue is to lower the amount of torque at each cadence RPM value; changing the torque slope by pivoting around the maximum cadence value. This lowers the power curve, but doesn't change the peak power cadence value - so at least we have that going for us.
A narrow optimum cadence range means we need lots of gears to be able to closely match wheel speed to cadence. This has been the trend for road bikes for years. Road bikes tend to be ridden for long periods at nearly constant speeds, the speed itself being limited by aero drag, the slope of the hill that is being climbed, or by how hard the leaders in the bunch are willing to work. With the speeds being constant, a road bike needs a lot of closely-spaced gears so that the rider can fine-tune his cadence (and thus power peak) to the current speed of the pack.
Road bike cassettes tend to be 10 or 11 cogs spaced one tooth apart to allow this fine tuning. The front chainring is used to select between a flat ground "big ring" and an uphill/high wind "little ring". There are exceptions - one can get cassettes with the last two or three ratios in bigger steps to provide "bail out gears" for very steep climbs - but for the most part, that is how a road bike is ridden: get up to speed in the pack, then trim to adjust cadence to the rider's power peak.
Mountain bikes are rarely ridden for long stretches at constant speeds. They might see some use like this on the road travelling to or from the trails, but once on the trail, the unpredictability and variability of the terrain means that the speed at which the bike can travel the trail is going to constantly change. More speed variation implies a requirement for a wider gear range.
The classic mountain bike gearing setup is a triple chainring (44, 32, 22 teeth) and a nine-speed rear (SRAM 990: 11, 12, 14, 16, 18, 21, 24, 28, 32). This provides a very wide range of gearing, and while the average 2-teeth steps on the rear cogs don't provide quite the cadence-trimming ability that a 1-tooth step road cassette does, neither is the need to trim cadence that exactly all that important - some power efficiency is traded for range.
SRAM XX adds a 36 tooth rear cog, providing an extra low gear. This extra low gear means that SRAM figures they can eliminate the granny (22t) chainring, and move to just two front chainrings (like a road bike). They provide three choices of front chainring sets so that the rider can match ratios to conditions.
A two-ring setup offers some benefit when compared to a three-ring. It is easier to design a derailleur that only has to make the one step in either direction, and chainring tooth profiles can be further optimised. The rear derailleur no longer needs to be able to accommodate the sudden change in chain length that happens with a double front downshift, so the chain can be shorter and tighter, which implies a reduced chance to drop the chain. And with only two chainrings to accommodate, the chainline can be improved so that the chain sees less angularity in plan view when run at the extreme ends of the cassette (with associated benefits in wear, drivetrain losses, and the risk of dropping the chain).
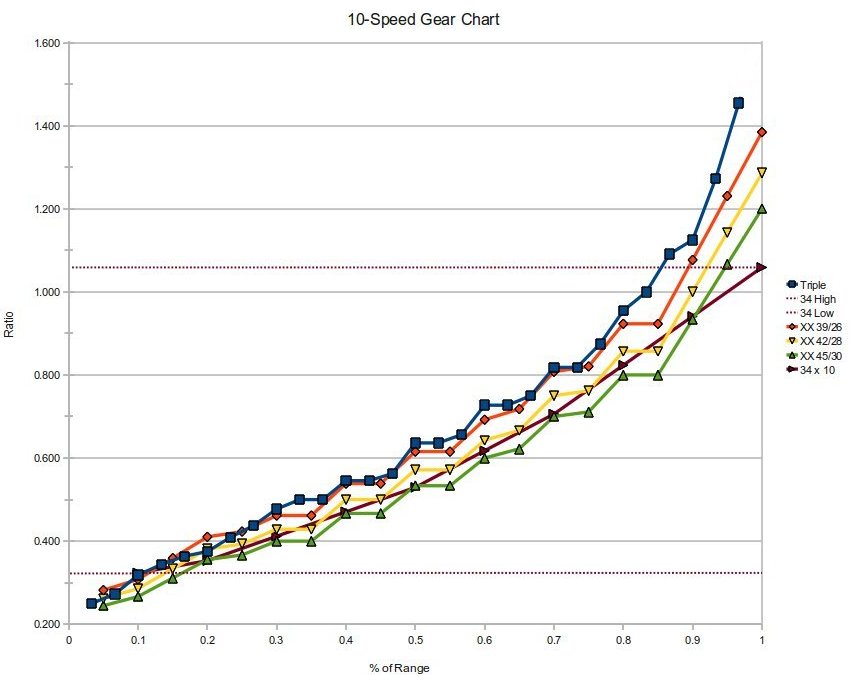
This graph charts the gear ranges of the classic triple setup, the three variants of SRAM XX, and a theoretical 34t single ring setup using the XX rear cassette. Higher values on the Y axis represent lower (easier) gears.
From this chart, we can see that the 39/26 XX setup gives up one gear (compared to 3 x 9) on the low side (it has a bull low lower than the second-lowest 3 x 9 gear, but it isn't quite as low as the 3 x 9 bull low), and two gears on the high side. XX 42/28 gives up one complete gear on the low side and one complete gear on the high side, and XX 45/30 gives away two complete granny gears but actually picks up some gearing on the high side.
The 1 x 10 with a 34t chainring gives away two gears on the high side (strictly speaking, it is three - but the 34t high is so close to the 44t third-highest as to make nearly no difference) and gives away three full granny gears (although you do get a bull low that is lower than the 3 x 9 32t low). Otherwise, it is identical is slope to the XX 42/28 setup.
Where this graph is misleading though is that it presents all the ratios from each setup as a linear progression of gears. That might be true in an electrically-shifted system like a Di2 Dura-Ace, where the computer knows what combination of front and rear gears provides the next ratio in sequence and makes the shift automatically (2011 Di2 doesn't do this yet, but someone has already hacked the controller to provide this function and Shinamo can't be that far away from doing it themselves) so unless the rider is willing to memorize a gear chart (and take the risk of a dropped front shift) and shift both shifters constantly, nobody will actually ride like this. It is worth looking at the gear ranges by chainring.
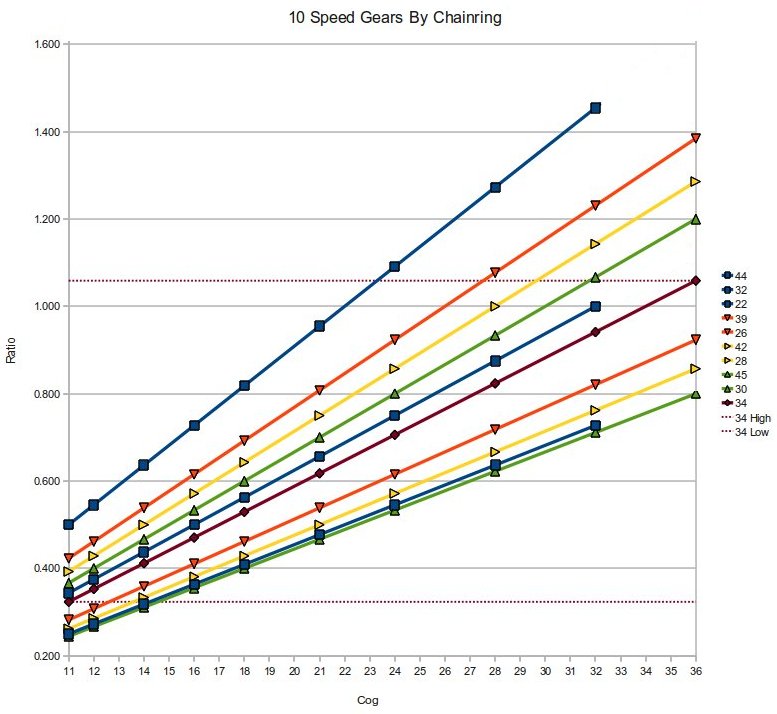
This view shows why XX works so well. There is a fair amount of overlap in each of their setups, so much like a road setup, you have a "hill" ring and a "flat" ring, and there's enough capacity in each so that the "hill" ring still works for a decent amount of flats and the "flat" ring still works for some hills. Plus, if a hill pops up unexpectedly, you can do a quick front shift and get a bunch of gear in a hurry (and the odds of dropping the chain are improved against a 3 x 9 setup)
The XX 42/28 covers nearly the same range as the 3 x 9 classic triple, or you can bias towards hill climbing or road riding by going with the other chaining combos.
But what I find to be very interesting is the 34t 1 x 10 setup. This setup gets you a bull low lower than the low gear on a 3 x 9 triple's 32t low that is nearly as low as the granny ring three-up low - meaning that you only lose two granny gears. But you pick up high end compared to the 32t and that gear is nearly equal to the 3 x 9's 44t three-down high. Depending on tire diameter and preferred cadence, that high gear should be good for 35km/h or so (which is road bike territory and well into the speed range where aero resistance is becoming a factor).
Based on the hills I typically climb (I did Alpe de Windsor in the 32t low; the big hill at Two Creeks is the 32t up-two low) and descend (Alpe de Windsor ran out of gear on the 32t high but I didn't want to risk the shift to the 44t) the 34t 1 x 10 looks to be ideal. I almost never use the top two gears on the 44t and I'm training myself away from the lower granny gears.
For a 1 x 9, the 34t front looks a little too big. I do use the 32t low gear and I wouldn't want to give that one up. My road bike uses a 50/34 compact crank and a 12-27 rear cassette. Given the larger size (29" vs 26") of the road bike wheels plus the 27t low gear, the 34 x 27 is roughly equal to the three-up gear on a 1 x 9. I've hit a couple of hills in the Meaford Area where that gearing was still a touch too high (at least at my current fitness level). I might be able to pull off a 34t 1x9, but it'd be good to have the 36t cog in my pocket for steeper stuff.
The final question is a chain retention device.
Because there is no need for the teeth to be profiled to assist shifting, a single chainring can use full-height teeth and that should assist chain retention. Plus, the chain can be run with less links and a short or medium cage rear derailleur which means less chain slack to take up, along with less bouncing around and other chain shenanigans. Nevertheless, it is a truth that the presence of the front derailleur helps prevent dropping chains when it is not being shifted. If the aim of going to a single chainring is to minimize dropped chains, then some sort of chain retention device is required.
The device I am most leaning towards is the Widget which uses a full-profile stainless steel chainring mated to a pair of thin, bashguard-esque chain guides. It is a little more expensive than the alternatives, but it offers chain retention features in both forward and backwards crank rotation - plus it is an elegant solution and I'm a fan of elegant.
As of right now, it is cost that is the single biggest showstopper to a 1 x 10 conversion. Converting means a new rear derailleur, a new rear cassette, a new rear shifter, a 10-speed chain, and the Widget.
In current prices (Nov 2010) the cassette is either $329 USD for XX (208g) or $250 USD for X0 (240g). The derailleur is $265 USD for XX, $234 for X0 (190g) or $118 for X9 (204g). Shifters can only be bought in pairs, and are $276 USD for XX (183g /pr) $238 USD for X0 (232g /pr), and $125 USD for X9 (232g /pr). The chain is $76 USD, and the Widget is $125 USD.
Depending on how you add that up, you are looking at $1071 USD for full XX or $694 for a mostly X9 system (and 95 extra grams) - clearly the X9 route is the way to go unless you can afford the bling of XX. That $700 buys you about a 450g weight savings compared to 3 x 9, which is actually on par for items like race wheels or carbon cranks.
I intend to - eventually - go down this route. Once I do, I'll post test results.
|
|